서 론
재료 및 방법
1. 실험 재료 및 SNP 처리
2. 광합성 측정
3. 단백질 정량 및 malondialdehyde(MDA), H2O2 함량 분석
4. 항산화효소 활성 분석
5. 생육 특성 조사
6. 통계분석
결과 및 고찰
1. 배추의 광합성 특성 변화
2. 배추의 MDA 및 H2O2 함량 변화
3. 항산화효소 활성 변화
4. 생육 특성 변화
서 론
배추(kimchi cabbage, Brassica rapa L. ssp. pekinensis)는 김치의 주재료로서 한국을 포함한 아시아 지역에서 널리 재배되고, 생산 및 소비가 많은 채소 중에 하나이다. 2022년 기준, 배추의 전체 재배 면적은 30,085ha, 생산량은 약 2,017천 톤이며, 10a당 생산량은 6,706kg이었다(KOSIS, 2023). 배추는 봄, 여름(고랭지), 가을 및 겨울 재배 등으로 작형이 세분화되어 연중 생산되고 있으며, 봄 재배는 전체 생산량의 34% 정도를 차지하고 있다(KOSIS, 2023). 특히 봄배추의 경우 육묘기에 저온에 의한 조기 화아 분화 위험성이 높고, 정식 후 고온 장일 조건에서 추대가 촉진되어 생산량이 감소할 수 있다(Lee 등, 2011; Lee 등, 2017).
식물이 저온 스트레스를 받으면 체내의 광합성이 줄어들고, 광합성 색소의 합성이 차단되어 광합성 효율이 감소하고 식물의 생장과 발달에 영향을 미친다(Short 등, 2020). 또한 식물이 과도한 활성산소종(reactive oxygen species, ROS)을 생성하고, ROS의 축적은 단백질, 지질, DNA 및 세포막 등을 손상시키며 다양한 생물학적 효소들의 활성을 저해한다(Baxter 등, 2014; Liu 등, 2010; Tang 등, 2021). 식물은 저온과 같은 환경 스트레스를 극복하기 위해 catalase(CAT), peroxidase (POD), superoxide dismutase(SOD)와 같은 ROS 제거 효소의 활성 증가와 malondialdehyde(MDA), 아미노산, 수용성 당(soluble sugar), 프롤린(proline)등의 축적과 같은 복잡한 대사체와 분자 조절 메커니즘이 작용한다(Caverzan 등, 2016; Mao 등, 2018; Mittler, 2017; Xie 등, 2019).
산화질소(nitric oxide, NO)는 신호전달 물질로서 식물 호르몬(옥신, 시토키닌, 지베릴린, 에틸렌)과 상호작용하여 식물체의 생장과 발달, 생리 반응, 기공 개폐 등을 조절하는 것으로 알려져 있다(Esim과 Atici, 2014; Neill 등, 2003; Zhao 등, 2008). 또한 NO는 가뭄, 염, 온도 스트레스, 병원체 또는 곤충에 의한 비생물적 및 생물적 스트레스에 대한 방어 반응에 관여한다고 보고되었다(Bellin 등, 2013; Fan과 Liu, 2012; Fan 등, 2013; Hayat 등, 2012; Kong 등, 2012). NO는 항산화제로 직접 작용하며, 삼투압 조절, 뿌리의 수송 시스템 활동의 강화에 의한 미네랄 영양분 증가, 지질막의 보호, 항산화효소 관련 유전자의 발현을 증가시켜 다양한 환영 스트레스 조건에서 산화스트레스를 완화시킨다(Fancy 등, 2017; Nabi 등, 2019; Oz 등, 2015; Saddiqui 등, 2011; Simontacchi 등, 2015; Yang 등, 2011). Sodium nitroprusside(SNP)는 식물체 내로 NO의 라디칼을 안정적으로 유지하면서 전달하는 공여체로, SNP 처리 시 오이(Yang 등, 2011), 밀(Esim과 Atici, 2014), 벼(Sohag 등, 2020), 배추 유묘(Fan 등, 2014) 등의 다양한 작물에서 저온 스트레스 저항성을 보인다고 보고되었다.
현재까지 배추의 환경 스트레스에 대한 SNP의 경엽처리 효과 연구는 미흡한 실정으로, 저온 조건 하에서 SNP 처리는 광합성 능력, 항산화효소 활성 등을 증진시킴으로써 스트레스에 의한 피해를 경감할 수 있을 것으로 기대된다. 따라서 본 연구는 이상기상 발생으로 인한 봄배추의 저온 조건 시 SNP의 처리가 배추의 생육 및 생리 반응에 미치는 영향을 구명하고자 수행하였다.
재료 및 방법
1. 실험 재료 및 SNP 처리
본 실험에서는 배추 ‘수호’(‘Suho’, Brassica rapa L. subsp. pekinensis (Lour.) Hanelt, Hungnong Seed Co., Korea) 품종을 사용하였다. 2021년 12월 2일에 시판용 바이오 상토 1호(Hungnong Seed Co., Korea)로 육묘용 플러그 트레이(72구, 540×280×45mm)에 충전 후 종자 1립씩 파종하였다. 이후 평균 기온이 25°C로 유지되는 온실에서 자연광 하에 육묘하였고, 본엽 6-7매가 전개된 모종을 사양토 12kg을 채운 와그너포트(1/2,000a)에 정식하였다. 정식 후 21일에 SNP(sodium nitroprusside, Sigma-Aldrich, Darmstatd, Germany)를 각각 7.5, 15 및 30mg·L-1 농도(SNP 7.5, 15 및 30)로 주당 100mL을 4일 간격으로 3회 엽면 살포하였고, 무처리(저온, Lt)와 대조구(적온, Ot)는 물을 처리하였다. 7일간 저온 조건(주간 16/야간 7°C)을 처리하였고, 종료 후에는 일반 조건(주간 22/야간 17°C)에서 재배하였다.
2. 광합성 측정
광합성 특성은 광합성 측정기(LI6800, LI-COR Inc., NE, USA)를 이용하여 SNP 엽면 살포 후 4일에 각 처리구당 6주를 대상으로 배추의 4번째 외엽에서 측정하였다. 챔버의 측정 조건은 기온 25oC, 이산화탄소 농도 400μmol·mol-1, 광도는 500μmol·m-2·s-1 PPFD로 설정하였다.
3. 단백질 정량 및 malondialdehyde(MDA), H2O2 함량 분석
SNP 경엽 처리 후 4일에 4-6번째 외엽에서 시료를 혼합 채취 후 액체질소를 사용하여 마쇄하였다. 각 시료 100mg에 1mL PBS(potassium phosphate buffer saline, pH 7.0)를 혼합하여 4°C, 13,000rpm에서 30분간 원심 분리 후 상등액을 추출하였다. Bradford(1976) 방법을 사용하여 단백질을 정량하였다. MDA와 H2O2 함량은 EZ-Lipid peroxidation(TBARS) assay kit(DoGenBio Co., Korea)와 EZ-Hydrogen Peroxide/Peroxidase Assay Kit(DoGenBio Co., Korea)를 사용하여 분석하였다(Lee 등, 2021).
4. 항산화효소 활성 분석
배추의 APX, CAT, POD 및 SOD 효소 활성은 각각 ascorbate peroxidase activity assay kit(Elabscience Biotechnology Inc., USA), EZ-catalase assay kit(DoGenBio Co., Korea), EZ-hydrogen peroxide/peroxidase assay kit(DoGenBio Co., Korea), EZ-SOD assay kit(DoGenBio Co., Korea)을 사용하여 분석하였다(Lee 등, 2021).
5. 생육 특성 조사
SNP 엽면 살포 후 32일(정식 후 54일)에 최대 엽장, 최대 엽폭, SPAD, 엽수, 엽면적, 생체중 및 건물중을 조사하였다. 엽록소 함량과 엽면적은 각각 SPAD 측정기(SPAD-502, Konica Minolta, Japan)와 엽면적 측정기(LI-3100, LI-COR Inc., USA)를 사용하였다.
6. 통계분석
실험구 배치는 난괴법 3반복, 통계분석은 SAS 프로그램(SAS 9.4, SAS Institute Inc., USA)을 이용하여 분산분석(ANOVA)를 실시하였다. Duncan의 다중검정을 이용하여 5% 유의수준에서 각 처리 간 유의성을 검증하였고, 그래프는 SigmaPlot 프로그램(SigmaPlot 12.5, Systat Software Inc., USA)을 이용하여 나타냈다.
결과 및 고찰
1. 배추의 광합성 특성 변화
저온 처리 시 배추의 광합성 속도는 전반적으로 감소하였으나, SNP 처리 시 무처리 대비 증가하는 경향을 보였다. SNP 처리 농도가 증가함에 따라 광합성 속도 또한 높아졌다. 특히 저온 처리 종료 후 회복 시점에서 SNP30은 23.5μmol CO2·m-2·s-1로 전체 처리구에서 가장 높았다(Fig. 1A). 또한 기공전도도, 세포 내 CO2 농도 및 증산 속도도 SNP 처리 농도에 따라 증가하였다(Fig. 1B-D). 식물이 저온에 노출되면 광합성 능력이 감소되며, 이는 단백질 합성 및 엽록소 생성 속도 저하, 틸라코이드막 지질 및 단백질 변화 등을 수반하여 일어난다(Bhattachrya, 2022). 환경 스트레스 조건에서 NO 처리 시 작물의 광합성 속도, 기공전도도 및 증산 속도가 증가하였으며(Alnusairi 등, 2021; Sardar 등, 2023; Sehar 등, 2023; Song 등, 2023), 높은 루비스코 활성을 통해 광합성 속도의 회복을 가속화하고(Sehar 등, 2023), 광합성 관련 유전자의 발현을 활성화시켰다(Xie 등, 2022). 저온 조건에서 SNP 처리 시 배추의 광합성 특성이 전체적으로 증가하였는데(Fig. 1), 이는 SNP 처리가 스트레스에 의한 광합성 효율의 감소를 효과적으로 완화시킨다는 것을 보여준다.
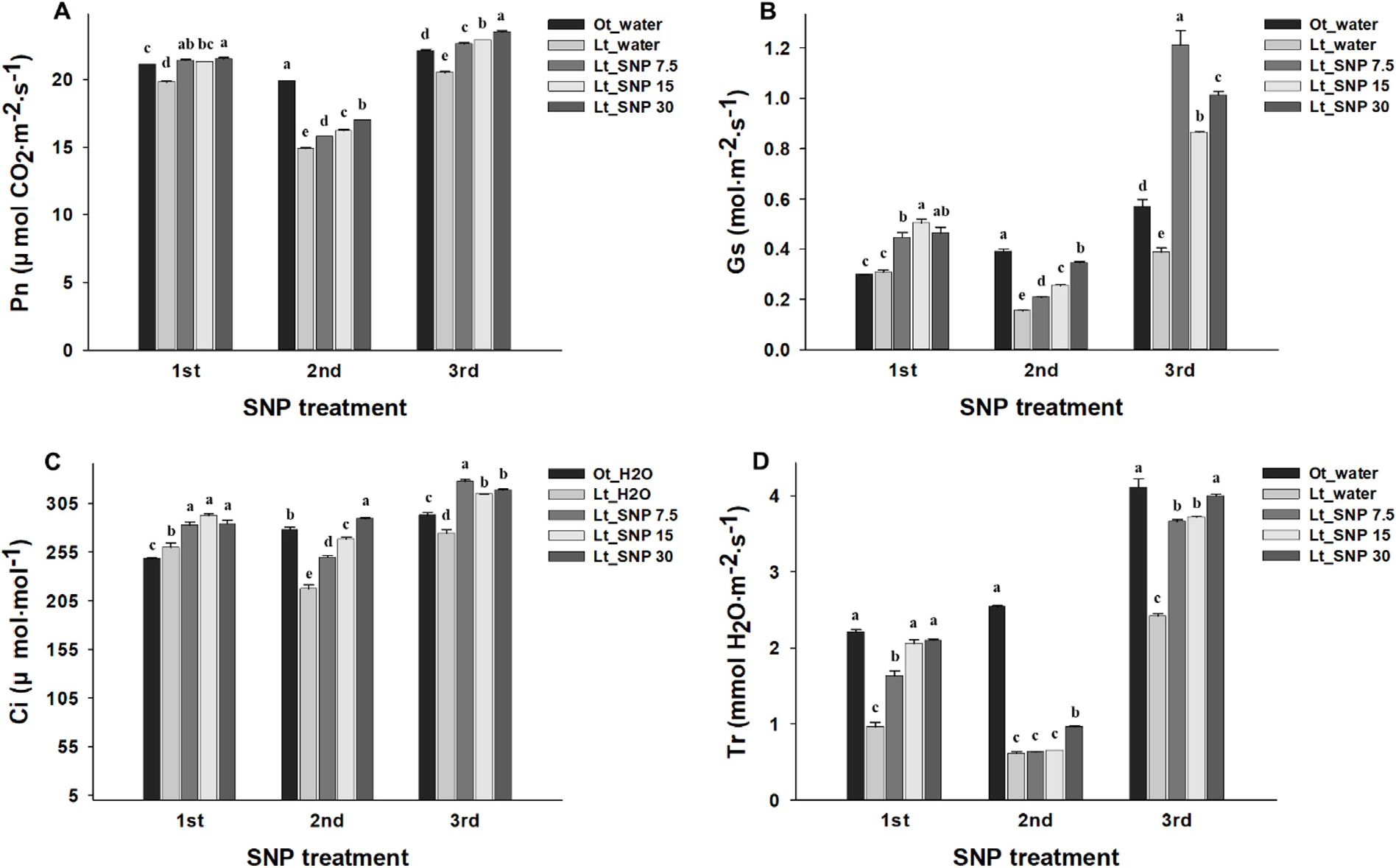
Fig. 1.
Comparison of photosynthetic rate (Pn, A), stomatal conductance (Gs, B), intracellular CO2 concentration (Ci, C), and transpiration rate (Tr, D) of kimchi cabbage leaves as affected by treatment with different concentrations of sodium nitroprusside(SNP) at 4 days after foliar application under low temperature condition. Vertical bars represent the standard error of means from six replications. Different letters indicate significant differences determined by Duncan’s test (p < 0.05).
2. 배추의 MDA 및 H2O2 함량 변화
저온 조건에서 SNP 처리 시 배추의 MDA 함량은 무처리 대비 1회 살포 후 28.4-32.2%, 2회 살포 후 9.4-12.2%, 그리고 3회 살포 후에는 23.6-28.6% 감소하였다(Fig. 2A). 반면 H2O2 함량은 2회 살포 후 감소하였으나 나머지 기간에서는 무처리 대비 증가하였다(Fig. 2B). 식물은 저온에 의한 스트레스를 받게 되면 세포 내 ROS 생성 등에 의한 대사작용 이상과 세포막 지질 분자의 과산화반응으로 세포막 파괴가 발생한다(Dhindsa 등, 1981). 이러한 환경 스트레스에 의한 세포 손상 정도는 MDA 함량으로 확인할 수 있는데(Chomkitichai 등, 2014), 저온 처리 시 무처리구의 MDA 함량이 대조구 대비 20% 이상 높았다(Fig. 2A). 반면에 저온 시 SNP 처리는 오이, 밀, 벼 및 유묘기 배추 등에서 세포 내 H2O2와 MDA 함량을 감소시켜 산화 스트레스를 완화하고(Esim과 Atici, 2014; Fan 등, 2013; Sohag 등, 2020; Yang 등, 2011), 세포 내 퍼옥시제나아제(poxygenase) 활성을 감소시켜 지질과산화와 세포 손상을 억제하는 것으로 알려져 있다(Zhao 등, 2008).
본 연구 결과, 저온 조건에서 SNP 처리 시 전반적으로 MDA 함량이 감소되었고, 처리 농도 간 차이는 없었다(Fig. 2A). 반면 H2O2 함량은 SNP 2회 엽면 살포 후 무처리 대비 감소하였으나, 나머지 처리 시기는 무처리 대비 증가하였는데(Fig. 2B), 이는 H2O2 함량이 항산화효소들의 활성에 따라 변화한다고 판단되며, SNP 처리는 저온 스트레스에 의한 세포의 손상을 효과적으로 완화시키는 것으로 사료된다.
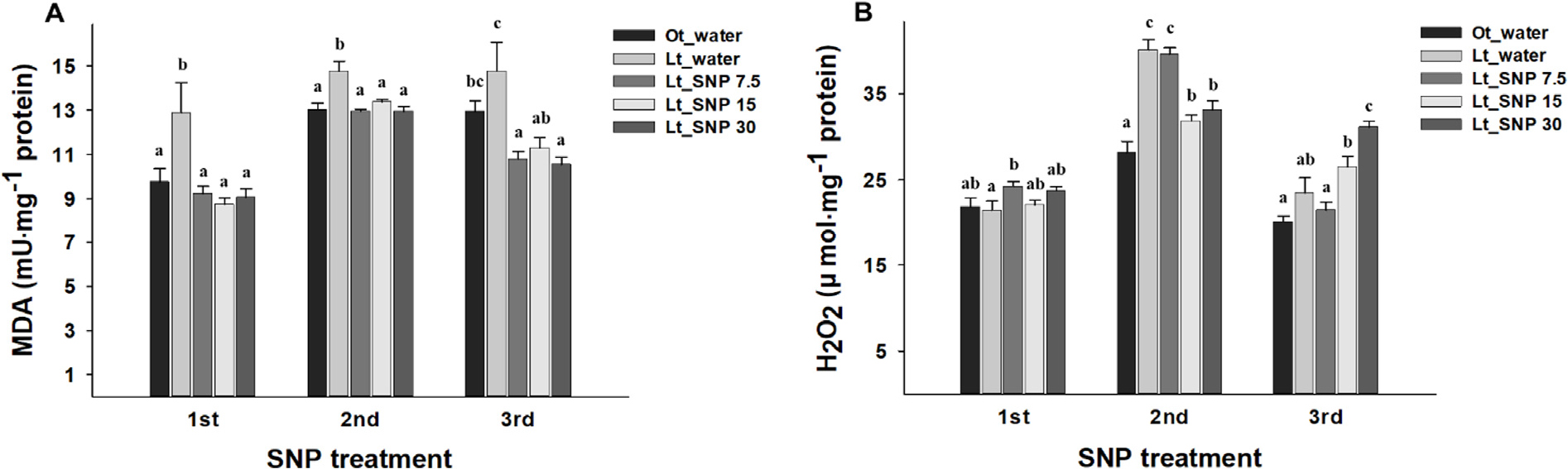
Fig. 2.
Measurement of malondialdehyde (MDA, A) and H2O2 (B) contents in kimchi cabbage leaves as affected by treatment with different concentrations of sodium nitroprusside (SNP) at 4 days after foliar application under low temperature condition. Vertical bars represent standard errors of the mean from nine replications. Different letters indicate significant differences determined by Duncan’s test (p < 0.05).
3. 항산화효소 활성 변화
저온 조건에서 SNP 처리 시 배추의 항산화효소 활성 변화를 분석하였다(Fig. 3). 저온 조건시 SNP 처리는 배추의 SOD, CAT 및 APX 활성을 증진시키는 반면에 POD는 처리 간 큰 차이를 보이지 않았다. SOD활성은 2회 SNP 처리에서 무처리 대비 2배 이상 높은 활성을 보였으며 특히 SNP15 가 가장 높았다(Fig. 3D). SNP15와 30 처리구는 전체 처리기간 동안 높은 CAT 활성을 유지하였으나, SNP 7.5 처리는 무처리구와 차이를 보이지 않았다(Fig. 3B). APX 활성은 1회 SNP 처리에서 무처리 대비 30% 이상의 높은 활성을 보인 반면 2회 처리 시 감소하였고, SNP30 처리구가 가장 높은 활성을 보였다(Fig. 3A).
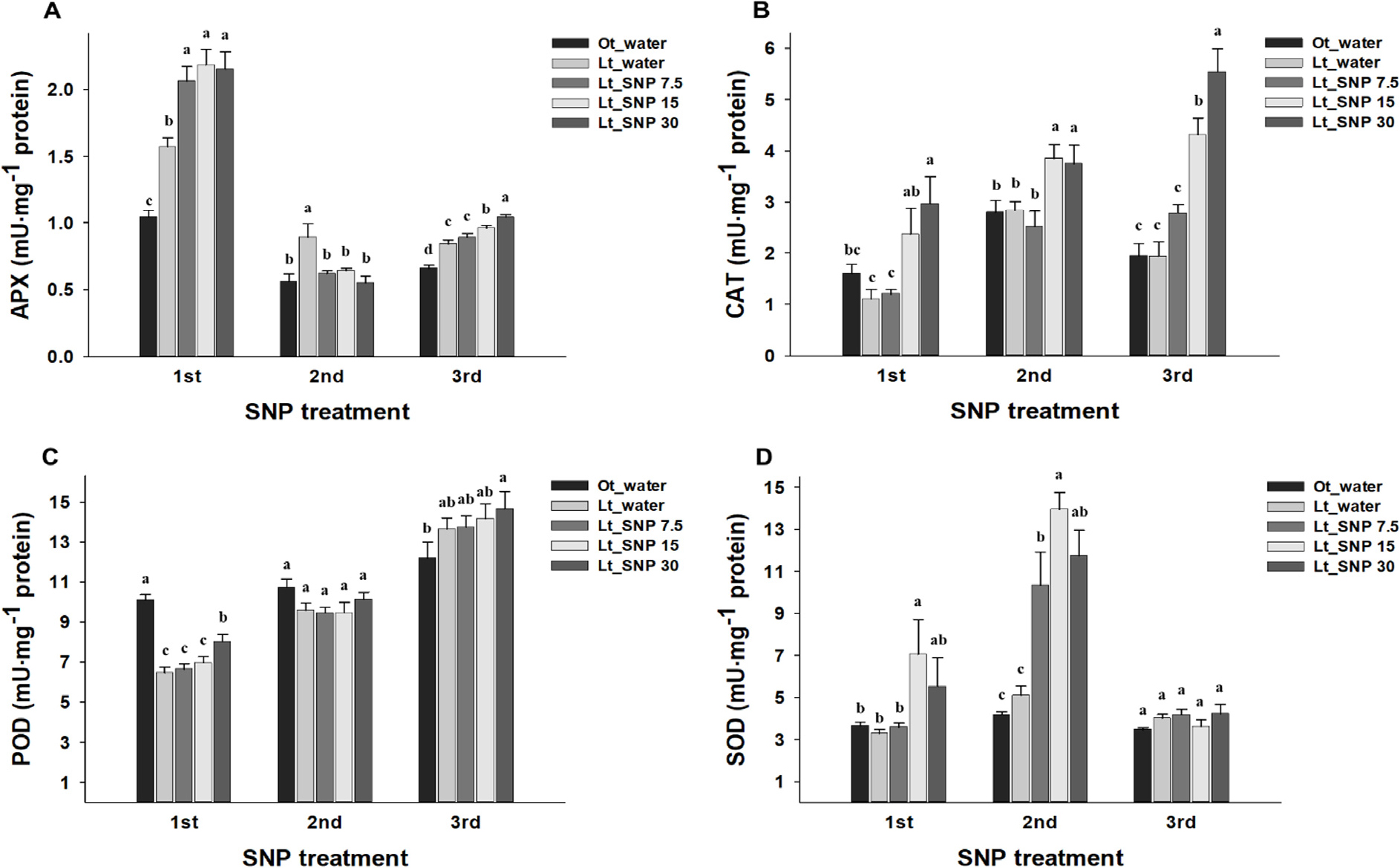
Fig. 3.
Comparison of ascorbate peroxidase (APX, A), catalase (CAT, B), peroxidase (POD, C), and superoxide dismutase (SOD, D) activity levels in kimchi cabbage leaves as affected by treatment with different concentrations of sodium nitroprusside (SNP) at 4 days after foliar application under low temperature condition. Vertical bars represent standard errors of the mean from nine replications. Different letters indicate significant differences determined by Duncan’s test (p < 0.05).
NO는 철을 포함하고 있는 항산화효소 활성에 많은 영향을 주고, 특히 CAT, APX 및 COX(cytochrome C oxidase) 관련 유전자의 발현을 활성화하며, 직접 과산화질산염 형태(peroxynitrite form)를 생성하면서 ROS를 제거하고, 세포 독성이나 손상을 유발하지 않는 것으로 보고되었다(Clark 등, 2000; Hayat 등, 2012; Liu 등, 2011). 이전 연구에서 일부 식물은 저온 처리 시 CAT활성이 감소하고, APX, POD 및 SOD 활성은 증가하는 경향을 보였고, SNP 엽면 살포 시 항산화효소 활성이 전반적으로 증가한다고 보고되었다(Fan 등, 2014; Sohag 등, 2020; Song 등, 2023).
본 연구에서는 저온 처리 시 APX 활성이 증가하고 나머지는 감소하는 경향을 보였으나, SNP 처리 시 농도 간 차이는 보이지만 전반적으로 항산화효소 활성이 증가하였다. 특히 APX와 SOD 활성은 저온 처리기간에서 가장 높을 활성을 보였다(Fig. 3). 또한 SNP 처리 시 항산화효소 활성의 변화는 환경 스트레스와 작물의 종류, 노출되는 생육 단계와 기간에 따라 영향을 받으며, SNP가 저온에 의한 산화 스트레스를 효과적으로 완화시킨다는 것을 보여준다.
4. 생육 특성 변화
저온 스트레스 조건에서 SNP 3회 엽면 살포 후 배추의 생육 특성을 조사하였다. 저온 처리 시 배추의 생체중, 건물중, 엽수, 엽면적이 감소하였다(Table 1). 이전 연구에서 저온 스트레스 조건에서 육묘 상태의 작물에 SNP 처리 시 생육 특성이 무처리 대비 현저하게 증가하는 경향이 보고되었다(Esim과 Atici, 2014; Fan 등, 2014; Sohag 등, 2020; Yang 등, 2011). 반면에, 본 연구에서는 SNP 처리구의 생육 특성은 무처리 대비 다소 증가하는 경향을 보였으며(Table 1), 이는 저온 조건에서 SNP 처리가 배추의 생육 저하를 다소 완화시키는 것으로 판단된다.
Table 1.
Kimchi cabbage growth as affected by foliar application of sodium nitroprusside (SNP) and exposure to low temperature condition at 54 days after transplanting.
Temperature | Foliar application |
Fresh weight (g/plant) |
Dry weight (g/plant) |
Leaf length (cm) |
Leaf width (cm) |
Chlorophyll (SPAD) |
Number of leaves (no./plant) |
Leaf area (cm2/plant) |
22/17°C | H2O | 489.5aZ | 30.1a | 30.6a | 17.9b | 41.8b | 52.3a | 6,266a |
16/7°C | H2O | 453.2b | 24.7a | 30.8a | 19.1ab | 45.9a | 47.9b | 5,817b |
SNP 7.5 mg·L-1 | 480.9ab | 29.0a | 30.8a | 18.6ab | 44.8a | 50.6ab | 6,184a | |
SNP 15 mg·L-1 | 472.3ab | 27.7a | 30.7a | 19.9a | 44.0ab | 49.9ab | 6,015ab | |
SNP 30 mg·L-1 | 478.2ab | 28.8a | 30.7a | 19.3ab | 44.6a | 50.1ab | 6,059ab |
따라서, 저온 조건에서 SNP의 경엽 처리는 배추의 광합성 능력을 향상시키고, MDA와 세포내 H2O2 함량을 감소시켰다. 또한 항산화효소의 활성을 증가시킴으로써 산화 스트레스를 완화하였고, 결과적으로 배추의 생육을 증가시켰다. 이 결과로 SNP가 배추의 생리적 활성을 증대하여 스트레스에 의한 피해를 완화시켰으며, 환경 스트레스 피해경감 기술로 활용될 수 있을 것이라 기대된다.